Navigating the Landscape of Green Jobs
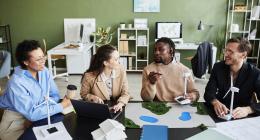
As batteries reach their power, weight and range limit, hydrogen appears to be a highly relevant fuel for decarbonising heavy-duty transport. However, some technological breakthrough is still needed to tackle on-board storage and energy efficiency issues associated with these vehicles.
Fuel cell electric vehicles (FCEVs) have short refuelling time, high range and low greenhouse gases (GHG) emissions when hydrogen is produced from renewable energy. In addition, pure hydrogen can be converted to hydrogen-based fuels such as ammonia, e-fuels and methanol which make it suitable for most transport uses (international shipping, trucks, buses etc.). The great advantages provided by hydrogen technologies come at the expense of energy efficiency losses. Further, due to its low energy density compared to conventional fuels, on-board storage volumes required for hydrogen may be an issue for long-distance transport.
Despite significant improvements in the range of battery-powered vehicles (BEVs) [1] and the growth of fast-charging stations, fuel cell vehicles have an undeniable technical advantage for long-distance transport. For BEVs, the volume and mass of batteries increases sharply as more energy storage is needed to satisfy their range and starting torque. However, the volume and mass of a hydrogen tank change only slightly as this need increases.
Figure 1. Comparison of fuel cell and battery vehicles, volume and mass required for energy storage, as a function of range. Sia Partners analysis based on data published in "International Journal of Hydrogen Energy" [2]
Further, as shown in the graph below, the International Energy Agency (IEA) expects hydrogen vehicles (FCEVs) to be more cost-competitive than BEVs for heavy duty transport with a range of over 500 km.
Figure 2. Total cost of ownership evolution of BEVs and FCEVs Ex: HEV $60/kw - Hydrogen vehicle with a fuel cell price set at $60 per kw. Sia partners analysis based on the IEA's "Energy Technology Perspective" report. [3] For this analysis, total vehicle ownership costs are calculated using different fuel cell and battery prices, assuming a constant hydrogen distribution cost of $5/kg and the same usage.
The use of hydrogen is particularly suited to the captive fleet concept. A captive fleet is a set of vehicles that depend on shared management and infrastructure (filling station and depot), whose relatively constant pathway allows a regular return to the filling centre. This is the case, for instance, for buses and commercial vehicles.
The enthusiasm for hydrogen buses is largely due to their range (about 300 km, allowing for a full day of service) and to their recharging time, limiting refuelling stops that interrupt battery-powered bus operations. As hydrogen buses have emerged gradually across Europe, France, the UK and the Netherlands have set ambitious targets for their deployment. In the UK, the first double decker was introduced in June 2021 and 3,000 hydrogen powered buses are expected to be in operation over the next 4 years. The Netherlands already has 60 buses on the road and aims to reach 3,000 heavy hydrogen vehicles in 2025 while France has deployed 30 hydrogen buses and several hundred are in the pipeline in about twenty French cities. Belgium, on the other hand, with around ten buses currently planned for the next few years, has less ambitious targets,.
European countries intend to use hydrogen opportunities to re-industrialise their economies. For this reason, buses are mainly ordered from national manufacturers. Northern Irish Wrightbus plants will deliver 3,000 hydrogen-powered buses for the UK. In France, Safra and Symbio have joined forces with a production target of 140 buses/year starting in December 2021. To support the development of a French industry, French energy companies Air Liquide, Engie and EDF (through its subsidiary Hynamics) are also involved in supplying service stations.
As the technology and opportunities for hydrogen within transport grow, concerns over how they will integrate within the current and planned infrastructure have arisen. Sia Partners studied the impact of the deployment of 1600 heavy hydrogen vehicles (high range French targets [4]) on the French national hydrogen production and renewable energy power generation capacities.
Heavy Road Transport | Consumption |
---|---|
Hydrogen consumption | 12kt |
Electricity consumption | 690 GWh |
Electrolyser | 3% France Capacity 2030 (200MW) |
PV capacity | 0,25% France Capacity 2020 (1,1 km2) |
Wind turbines capacity | 0,26% France Capacity 2020 (30 wind turbines) |
CO2 emissions avoided | 35 kteqCO2 |
Figure 3. Annual consumption and externalities of French heavy road transport. Sia Partner study.
Our analysis indicates that the deployment of hydrogen vehicles for road transport would use 3% of the installed electrolysers capacity in France and would avoid 35kteqCO2 annually.
90% of the world's trade is carried by ships, contributing for 2-3% to the world's greenhouse gas (GHG) emissions. Compared to other modes of transport, there are fewer alternatives for the decarbonisation of shipping. According to the IEA [5], the use of batteries in the maritime sector is restricted to ranges of around 200 km, which represents a small fraction of all maritime routes. Additionally, whilst blending biofuels with fossil fuels could facilitate the gradual decarbonisation of the sector over the short and medium term, it will face supply limitations in the longer term. The supply limitation is driven by the impact of climate change on crop yields and the growth of biomass uses - for food, energy, biogas production, bioproducts, biofuels, etc. – which are already hampering the future perspective of this energy source.
In comparison, hydrogen holds great potential for the maritime sector, however the advantages differ by fuel type and depend on the use and distance covered. Ammonia has been identified as a feasible alternative fuel for the decarbonation of maritime transport. Made from hydrogen by well-proven industrial processes, ammonia has many advantages over hydrogen for ship fuelling, such as a greater energy density, the ability to adapt to current ships with few modifications, and easier transport/storage conditions. In addition to not emitting carbon (CO2, CO) during its combustion, ammonia also has low production, storage and transport costs compared to other low-carbon fuels.
Fuel | Advantages | Disadvantages |
---|---|---|
Liquid Hydrogen | Liquid hydrogen has higher energy density than gaseous H2 thus needs less storage space. There are no leakage problems related to storage in gaseous form. This is a very powerful decarbonation lever for long-distance transport on roads with frequent refuelling. | However the liquefaction process is energy intensive. It also requires more storage space than other low carbon alternative fuels. In addition, liquid hydrogen is very flammable in this form and requires highly insulated tanks (liquid hydrogen at -253°C at 1 bar). |
Gaseous H2 | Gaseous hydrogen has low cost processing and few energy losses. | It possesses low energy density thus the volume of storage systems are too large for long distance. Storage conditions are also particularly demanding. (700bar at 20°). |
Ammonia | The energy density is twice as high as liquid hydrogen and combustion in existing engines is possible with only slight adaptation Ammonia is also easily stored (10 bar at 20°C) and possesses similar usage properties to LPG thus there is the possibility to use it in existing storage/transport infrastructures. | It requires additional chemical transformation then energy efficiency is pretty low. Ammonia also corrodes storage systems and is toxic for human health. Further, CO2 footprint is higher than hydrogen on full life cycle (refer to figure 4). |
IEA foresees the use of hydrogen in gaseous or liquid form for short and medium distance shipping. However, for long-distance transport, hydrogen must be liquefied to increase its volumetric density. Because of its technical constraints, IEA predicts a preferential development of ammonia, which accounts for the majority of the long-term supply of the maritime sector in its 2020 Sustainable Development Scenario (SDS).
While ammonia confirms its value from a consumer perspective, its greenhouse gas (GHG) emissions are slightly higher than those of hydrogen over its entire life cycle.
Figure 4. Comparison of various marine alternative fuels. Emissions over the whole life cycle, from extraction to combustion. (Assumption of electrolysis from 100% ENR mix) [6]
Hydrogen and ammonia are, therefore, of interest for decarbonation on the condition that they are produced by low-GHG emission technologies, through electrolysis using low-carbon electricity or through steam reforming combined with carbon capture solutions.
Figure 5. Mapping of marine hydrogen projects. Sia Partners.
Hydrogen-based fuels, namely pure hydrogen and ammonia, are already driving the shipping industry's efforts to decarbonate international maritime trade. The specific requirements of this transport mode could be one of the major outlets for the hydrogen industry.
Sia Partner has studied the impact of the deployment of 100 heavy containerships (hydrogen industry targets [7]) on the French national hydrogen production and renewable energy power generation capacities.
International Shipping | Consumption |
---|---|
Hydrogen consumption | 780 kt |
Electricity consumption | 43400 GWh |
Electrolyser | 190% France Capacity 2030 (12 500MW) |
PV capacity | 15,6% France Capacity 2020 (69 km2) |
Wind turbines capacity | 14,4% France Capacity 2020 (1670 wind turbines) |
CO2 emissions avoided | 4400 kteqCO2 |
Figure 6. Annual consumption and externalities of international maritime transport in France. Sia Partners analysis.
Our analysis shows, under the assumption that hydrogen is produced exclusively from renewable energy, France would have to import at least half of the hydrogen consumed by its fleet in 2030. This is mainly due to the low efficiency of electrolysers connected to renewable energy sources, that could be compensated by a connection to the grid. This conversion would avoid 4.4 MteqCO2 each year compared to heavy fuel oil
Although hydrogen is a relevant energy vector to reach the European objectives for reducing GHG emissions from transport by 60% by 2050, the lack of production, transport and distribution infrastructures challenges its competitiveness. In the short term, the construction of refuelling stations to accommodate captive fleets would make it possible to develop the preliminary infrastructures for road transport. Moreover, hydrogen and ammonia could play a key role in meeting the European Commission's target of a 40% reduction in shipping’s GHG emissions, even though efforts are still needed to be economically competitive with other carbon-based equivalents.
The urgent need for substantial structuring of these sectors offers an opportunity for the players in the sector such as manufacturers, energy companies, hydrogen suppliers, hydrogen equipment manufacturers, etc. to lead the way forward. Sia Partners, through its expertise on hydrogen, is willing to support the key players in this transition.
[1] Range of 800km reached by the Tesla Model S sedan or the Tesla Semi truck.
[2] Thomas, C. E. (2009). Fuel cell and battery electric vehicles compared. International Journal of Hydrogen Energy, 34(15), 6005–6020.
[3] AIE, Energy Technology Perspectives 2020
[4] High range objectives of the French Pluriannual Energy Program. Hydrogen produced from a 100% renewable energy mix. The following parameters were used:
[5] From AIE, Energy Technology Perspectives 2020. Hydrogen price used: 5 $/kg.
[6] P. Gilbert, Assessment of full life cycle air emissions of alternative shipping fuels, Journal of cleaner production, October 2017
[7] Targets for the French hydrogen sector (Afhypac). Assumption of 10% of the French boat fleet converted for international transport. Hydrogen produced from a 100% ENR mix. Following parameters have been used: